Construction is underway for the newest facility at the U.S. Department of Energy’s (DOE) Brookhaven National Laboratory. The Science and User Support Center (SUSC) is the first building for the planned Discovery Park, a development the Laboratory is pursuing near its entrance along William Floyd Parkway.
The three-story, 75,000-square-foot facility will serve as a welcome center for the 75-year-old Brookhaven Lab, which is home to seven Nobel Prize-winning discoveries and hosts thousands of guests annually. The SUSC will also offer conference and collaboration areas for scientists as well as office space for the Lab‘s support staff.
Officials from DOE and Brookhaven Lab commemorated the start of construction during a groundbreaking ceremony Wednesday, Oct. 26.
DOE’s Under Secretary of Science and Innovation Geri Richmond said, “This strategy—of welcoming the community to be part to our nationallaboratories and focusing on creative, innovative ways for public-private partnerships to strengthen the economy—is so important. This is a centerpiece, a catalyst for the campus and for the future.”
Manager of DOE’s local Brookhaven Site Office, Robert Gordon, said, “This is transformative for Brookhaven National Laboratory. We should be accessible. We’ve done that with our words and our actions. Now we’re doing it with concrete.”
Brookhaven Lab Director Doon Gibbs said, “This construction is a milestone in the Laboratory‘s long-term strategy to revitalize its physical plant. We look forward to welcoming visitors, users, students, and members of the community to connect with Brookhaven, the DOE, our science, and the impact it has.”
Plainview-based E.W. Howell is leading construction as the project’s general contractor. The Laboratory announced in February that it awarded E.W. Howell a $61.8 million contract to build the SUSC. DOE approved a total cost of $86.2 million for the project. E.W. Howell and BrookhavenLab are targeting 2024 for construction to be completed.
The SUSC is the first building planned for Discovery Park, a new vision for Brookhaven Lab‘s gateway with approximately 60 acres of previously used, publicly accessible land. The Laboratory is working with DOE on a process for developers, collaborators, and entrepreneurs to propose, build, and operate new facilities that could complement DOE and Brookhaven Lab‘s missions and leverage opportunities from close proximity to the Laboratory.
Empire State Development is supporting Brookhaven Lab‘s efforts for Discovery Park with a $1.8 million capital grant, recommended by the Long Island Regional Economic Development Council.
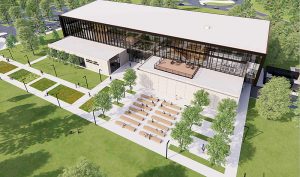
Increasing Efficiency for Discoveries, New Technology
Brookhaven Lab attracts scientists from across the country and around the world by offering expertise and access to large user facilities with unique capabilities.
Brookhaven hosted more than 4,400 in-person and virtual scientists from universities, private industry, and government agencies in fiscal year 2021. In the years before the COVID-19 pandemic, more than 5,000 guests and facility users visited each year. The Laboratory expects the number of guests researchers to increase in the coming years, particularly as capabilities expand at the National Synchrotron Light Source II—a DOE Office of Science User Facility—and with the design and construction of the future Electron-Ion Collider.
The SUSC, when complete, is where those guests will arrive. The SUSC will also help improve the guests’ experiences of visiting Brookhavenbecause the Laboratory will consolidate a number of guest services into a central, modern building close to the site entrance.
The SUSC will also feature reconfigurable conference space, designed in response to requests from facility user communities to create opportunities for scientists to collaborate.
In addition, the SUSC will help the Laboratory increase efficiencies by reducing its building footprint atop the 5,322-acre site. The Laboratory plans to relocate approximately 225 staff at the SUSC. They are currently spread across the Lab site, which contains 314 buildings—some that date back to the World War II era, when the Laboratory was the site of the Army’s former Camp Upton.
The SUSC project is funded by the DOE Office of Science.
Brookhaven National Laboratory is supported by the Office of Science of the U.S. Department of Energy. The Office of Science is the single largest supporter of basic research in the physical sciences in the United States and is working to address some of the most pressing challenges of our time. For more information, visit science.energy.gov.