Life Lines: The origins of multicellularity
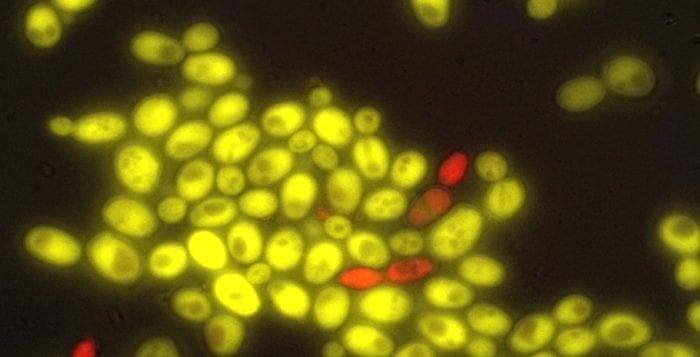
By Elof Axel Carlson
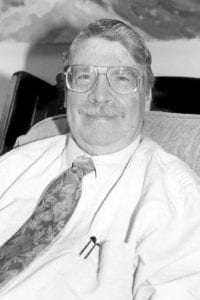
You are a multicellular organism. In fact you have about 37 trillion cells. That’s 37,000,000,000,000 if you like numbers. Cells were first described in 1665 by Robert Hooke who looked at cork bark under a crude microscope he had invented. The cells he saw were empty boxes. He believed this was the cause of cork’s buoyancy.
It wasn’t until the mid-1800s that lenses and stain technology developed to reveal detail inside cells. It also permitted several biologists to promote a theory that all cells arise from pre-existing cells and that organisms are composed of cells.
In that stage of our knowledge of life, scientists worked out mitosis (how cells divide) and meiosis (how cells form reproductive cells). They learned that chromosomes carried the genes, or hereditary units, that produce all the components and cellular types in an organism. In the last half of the 20th century they learned how to take apart and put together components of the living cell.
We see multicellular life among plants and animals around us, but we cannot see single-celled organisms without a microscope. Microscopic single cells exist for bacteria, certain algae, certain fungi and most protozoa. The presence of multicellular organisms goes back to about 2.5 billion years ago with filaments of cells in ancient rocks.
About 20 years ago I was delighted to read about experiments by Nicole King (UC Berkeley) showing that one-celled organisms, similar to those found in sponges, could be selected to join in clumps. That has been greatly extended to algal cells (Chlamydomonas, Volvox) and fungi (yeast).
William Ratcliff at Georgia Tech recently published results of selection for larger and heavier yeast cells that settled down on the bottom of test tubes. He isolated some that developed adhesions. From continued selection (hundreds of generations of yeast) he obtained some that formed flakelike arms or branches and that reproduced by breaking off branches.
King, who continues her work, has isolated more than 300 genes associated with multicellularity, many of them found in single-celled organisms. By combining different groups of genes, she can increase the likelihood of producing multicellular units.
Multicellular organisms can be simple like balls or they can be complex with specialized tissues and organs. They can dig deeper into the earth or extend their range from a few feet to miles or across continents. There have been millions of species that constantly change the way the surface of the earth appears. We are now in a molecular age in which individual genes can be sequenced and their functions studied.
If I see a picture of myself, I see my surface of skin and hair clothed or unclothed. With X-rays I can see my bones, but not as well as a human skeleton mounted in an anatomy laboratory. I have seen what my tissues look like from a box with a hundred or more slides that I studied at NYU as an undergraduate.
I have lived through the discoveries of identifying my genes as made of DNA, and we are now capable of sequencing them and understanding what they do. Each finding adds to both our medical knowledge for pathologists and to basic science in understanding how a living organism works.
I would not be surprised to see experiments that will produce synthetic multicellular organisms using genes from different organisms to produce differentiated cells for each task desired. It will be a biological engineering that goes beyond applications to the pharmaceutical industry. Think of them as microscopic or miniature tools. Imagine tools snipping away tumors less than a millimeter in diameter. Imagine such tools extracting and expelling miniature pellets of gold and rare metals from ocean water.
For those who worry about unintended consequences of applied science, two things are important to consider. Such experiments should be well regulated by ethical and safety review boards by universities, hospitals and corporations. The odds of such synthetic organisms are remote. Similar safety concerns in the 1980s accompanied the development of genetically modified bacteria and yeast cells, which today continue to produce human insulin for diabetics, human growth hormone for children with pituitary hormone deficiencies and hundreds of other modifications.
Elof Axel Carlson is a distinguished teaching professor emeritus in the Department of Biochemistry and Cell Biology at Stony Brook University.