By Daniel Dunaief
Decades ago, most people could only tune to shows like The Jetsons to imagine interactive televisions in which people could see each other during conversations.
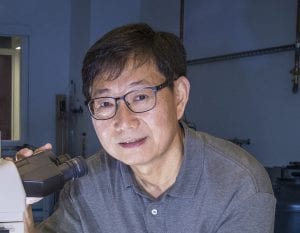
In modern times, hand held devices and laptop computers have turned those science fiction ideas into everyday realities, as people can tell their phones to call their mom, to provide the outdoor temperature or to help them recall the name of a movie they saw decades ago.
These helpful technological devices, however, may some day go the way of the clunky desktop computers of yesteryear, as scientists around the world work to turn the vision of a quantum computer into a reality.
Scientists hope to develop a next generation of quantum computer that is faster, smarter, more flexible and more energy-efficient than current technological devices. They hope these devices could be the key to future technological breakthroughs, inspiring them to figure out how to bring the theory to life.
Collaborating with scientists at Ames Laboratory in Iowa, Qiang Li, SUNY Empire Innovation Professor in the Department of Physics and Astronomy at Stony Brook University and Leader of the Advanced Energy Materials Group at Brookhaven National Laboratory, recently published a study in the journal Nature Materials that provided fundamental information that might contribute to the field of quantum computers.
The group of scientists, which included Li’s PhD student Pedro Lozano, discovered a light-induced switch that twists the crystal lattice of a semimetal, turning on an electron current that the team believes is nearly dissipationless.
When currents move through wires between utilities and people’s homes and offices, that current encounters resistance, losing energy along the way, as if the movement towards the home created a tax on the journey. Similarly, dissipation inside an electronic device can sap some of the energy needed to transmit information or a signal, reducing the effectiveness of the process.
Li and BNL physicist Genda Gu synthesized, patterned and characterized the material at BNL, while Jigang Wang, a senior scientist at Ames Laboratory, performed the light-induced lattice twisting. The team helped create the light-induced switch.
Li described the effort as “fundamental research” and cautioned that any such advancement is more of a principal study, rather than a step closer to making any new qubit (the basic unit of quantum information) device.
“This is an experimental study to show that this is possible,” Li said. “It’s a demonstration of feasibility that you can harness chirality for building quantum information systems.”
With chirality, electrons have a handedness based on whether their spin and momentum are aligning in the same or opposite direction.
Once electrons have chirality, they can travel much easier, enabling a more direct and predictable route from one place to another.
Scientists like Li would like to create physical systems that enable them to control the chirality, preventing the spin from switching from one direction to the other.
Numerous factors can disrupt the chirality of an electron, including imperfections in the material.
A pulse-triggered light-induced switch can change the topology of a Weyl semimetal, making it possible to enable the movement of electrons that are nearly dissipationless. “For pure electronics, even computer chips, electrons consume a lot of energy because of electrical resistance,” Li said. “A chiral current [however] will travel without resistance, in ideal cases without chirality flipping.”
Chiral electrons travel through the semimetal at a speed as high as 1/300th of the speed of light and can travel considerably further before a collision that alters its direction, speed, or other particle properties. The mean free path, which is the average distance a particle will travel between such disruptive events, for a typical metal is nanometers. By contrast the chiral electrons can move micrometers, which is thousands of times longer.
An unperturbed chiral electron could travel further distances over shorter intervals, carrying preserved coded information without losing much energy during movement.
Scientists have sought ways to create a path through which electrons travel with this predictable spin. They can break chiral symmetry by applying a magnetic field, which led to the discovery of the chiral magnetic effect by a team of scientists from BNL and Stony Brook University, including Dmitri Kharzeev, in 2014.
For this work, Li received the Brookhaven Science and Technology Award in 2019.
“Using a magnetic field is problematic for some computations,” he said. Besides, people don’t want a “big magnet around your computers.”
Another way is to send in the laser pulse, creating left-handed or right-handed polarization.
To determine the ideal pulse to change the material, Li and Wang partnered with several theorists from Ames Lab and Ilias Perakis, Professor and Chair of Physics at the University of Alabama — Birmingham.
The theorists conducted detailed analysis of the lattice vibrations and the ideal pulse energy needed to break symmetry in the Weyl semimetal. “There is a very strong collaboration between the theorists and the experimentalists,” Li said.
While the research remains fundamental and is unlikely to generate a specific product any time soon, Li said it has “attracted a lot of attention” from other scientists and is a significant step forward in establishing the basic principles for topology-enabled quantum logic and information systems.
Li and Wang have been collaborating on this project for about two years as scientists around the world are in a “horse race” to produce results in the arena of quantum computing.
A resident of Setauket, Li and his wife Meiling Shih, have two children. Shih, who worked in the Stony Brook Pharmacological Science Department and later at Morgan Stanley, is retired and is now a volunteer instructor of a Tai Chi class for local seniors,
Li enjoys jogging and runs a few miles every other day.
Down the road, Li hopes to address how to make the two quantum bits talk to each other.