By Elof Axel Carlson
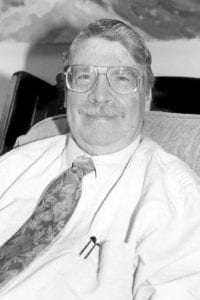
In 1997 I sent Leah Dunaief several essays that I called Life Lines and I asked her if she thought these might be of interest to the readers of her new newspaper, the Village Times.
About a month later she replied and said she retrieved my packet from her pile of correspondence and liked my essays and thus began a 25 year association and more than 400 columns of Life Lines as her newspaper chain grew in number across the North Shore of Long Island.
My aim was to inform my readers of the importance of science in our lives. I am a professor, now retired, who taught in Canada (Queen’s University), UCLA, and Stony Brook University as well as holding visiting professorships over the years in San Diego State University, the University of Utah, the University of Minnesota, and Tugaloo College in Mississippi.
I have also taught twice on Semester at Sea a floating campus that sales eastward or westward around the world for a semester with 500 students. I have taught also in programs for a federal program to raise science literacy in former USSR republics, including Samara — on the Volga River, and Tbilisi in former Soviet Georgia. I have mentored 6 students through their PhDs in my laboratories and have published 15 books on genetics, the history of genetics, the eugenics controversy, and science and society conflicts. I love to teach and have taught thousands of students at UCLA and Stony Brook University in a course titled Biology 101-102 Biology – A Humanities Approach.
I argued that a knowledge of science and its history and its application to society is important in showing how science has greatly reduced infant mortality, greatly increased life expectancy, eliminated most communicable disease, and restored health to millions of people through its applications to our food industries, pharmaceutical industries, and public health programs.
It gives us greater control over our lives and allows a person like me to live more than 90 years. I will be celebrating my 91st birthday in July and I am grateful that I have lived an examined life, avoided alcohol and other harmful agents in my life and have insights into how life works that are worth sharing.
I read widely, love the arts and humanities, consider science to be part of the liberal arts education we should extend past K-12 to college level programs that make us informed citizens in a democracy and parents able to make informed decisions about their children’s well-being rather than relying on political ideologies or religious traditions first introduced two or three thousand years ago as guides for how to protect ourselves and our families.
Science allowed me to understand how life works. I can follow it from atoms to molecules, macromolecules, cell organelles, cells, tissues, and organs. From there I can extend it to the population, the diversity of life on earth, and the importance of human stewardship of that life by informed ways using the earth’s bounty, protecting it from pollution, avoiding erosion, preventing desert formation, and preventing discharges of carbon dioxide from the fuels we use that lead to climate change that can flood our coastal cities and bring chaotic weather patterns around the world.
I thank Leah Dunaief and her son Daniel for the many articles on science that inform North Shore readers of what is going on in our universities, research institute, and industries on Long Island. I thank you readers who have written to me, stopped me when I shopped in the local stores, or offered different points of view to which I would respond.
I have lived an examined life, selected, as an Epicurean, from the best minds and writing of Western civilization. I am grateful for the gift of life to have lived this long. But now in my 90s I lack the energy I had ten years ago and have decided to use the time remaining in my life to work on my unpublished manuscripts and get them published or place them to print-on-demand programs on the internet. I much appreciate you, my reader, for the pleasure of having this opportunity,
Elof Axel Carlson